We are delighted to announce the finalists for the James F. Crow Early Career Researcher Award! All finalists will speak at the Crow Award session of the Population, Evolutionary, and Quantitative Genetics Conference on May 14, 2018 in Madison, Wisconsin.
The Award honors the legacy of James F. Crow, whose contributions to the field of genetics were impactful and innumerable. Learn more about Crow and the award.
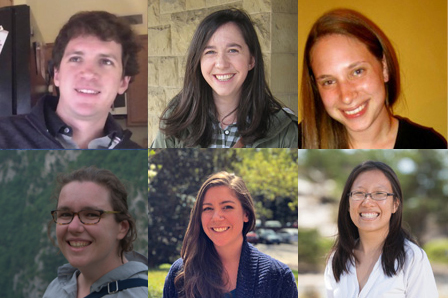
Crow Award finalists: Top row, left-right: Jeremy Berg, Alison Feder, Amy Goldberg; bottom row, left-right: Emily Josephs, Emily Moore, Katherine Xue
Jeremy Berg
Columbia University
I have always been interested in how things work. As a child, this manifested first as an interest in understanding how various pieces of machinery worked, and later in the laws of physics and cosmology. As an undergraduate student at the University of Wisconsin (where I was fortunate enough to have Crow as a guest lecturer a few years before his death), my interests turned toward the biological, and particularly to evolution. It seems natural then that I was drawn to population genetics, as it is the science which deals on a mechanistic level with understanding how the evolutionary process works, and I chose to pursue a PhD in this area at UC Davis.
In particular, my work focuses on understanding how evolutionary processes played out over the past ~200,000 years have contributed to the generation of diversity within the human population. Sometimes, this takes the form of understanding recent adaptations that have evolved within human populations, which gives us an insight into the challenges that our ancestors faced and the kind of traits that helped them survive and prosper. Another branch of my work focused more on the role of evolution in genetic disease, and in particular understanding why certain genetic diseases persist in the population despite their obvious fitness costs, and what forces are respsonsible for shaping their underlying genetic architectures.
Go to Jeremy Berg’s presentation abstract.
Alison F. Feder
Stanford University
I loved both math and life sciences classes throughout school, so when I started college, I sought out a research experience in quantitative biology. This search led me to Warren Ewens and Joshua Plotkin, who showed me how quantitative approaches could be especially instructive in understanding evolutionary biology. For example, when a population changes over time, can we use statistics to determine whether chance or selection drives those changes? This research question, which I pursued in Dr. Plotkin’s lab as an undergraduate, seeded a longstanding interest in understanding adaptation quantitatively.
I want to understand how and when populations adapt in the face of daunting evolutionary odds. Whereas most empirical and theoretical work has considered populations evolving to a single challenge, in nature, these challenges often are multiple and sometimes orthogonal. For example, we currently treat HIV with combinations of three drugs that attack different stages of the viral life cycle. This treatment strategy has substantially reduced but, importantly, not eliminated, the evolution of drug resistance within patients. How does adaptation still occur under such improbable conditions? I use HIV as a case study to understand evolution to selective pressures of varying complexity, including those structured in physical space. Many natural and man-made environments present similarly difficult challenges to species in nature. What separates the conditions that allow some species to persist via adaptation but drive others to extinction? Long-term, I hope to address such questions in a way that both improves human health and advances our fundamental understanding of evolution.
Go to Alison Feder’s presentation abstract.
Amy Goldberg
University of California, Berkeley
The recent availability of large high-coverage genetic datasets, combined with careful ecological sampling, has demonstrated the outsized impact that recent evolutionary history—the last tens of generations—can have on the genetic and phenotypic variation of a population. I develop quantitative methods to elucidate recent population histories, and interpret these histories in the context of demographic, cultural, and environmental pressures. Starting my scientific career with archaeological fields schools, my strong interest in mathematics and a desire to quantitatively test hypotheses drew me to population genetics.
Admixture, or hybridization, is one of the fastest evolutionary processes to radically change the composition of a population. Admixed populations are formed through the exchange of individuals from two or more previously isolated populations. Acting as a natural experiment, admixed populations offer insight into adaptations of their parental populations, and are ubiquitous throughout animal and plant populations. Multiple processes such as inbreeding avoidance and phenotypic mate preferences direct how parental populations mix. Despite this complexity, previous methods often considered admixed populations as simple instantaneous combinations of their sources. Instead, my work takes a mechanistic approach, deriving flexible sets of mathematical models to consider the admixture process, which often varies in intensity geographically or over time. Importantly, my theoretical results demonstrate that previous work—which does not consider sex bias, nonrandom mating, or the mechanistic process—misestimates population history parameters, such as the timing and intensity of migration. My work also links admixture dynamics with classic population genetic models of breeding and assortative mating by genotype.
Go to Amy Goldberg’s presentation abstract.
Emily B. Josephs
University of California, Davis
I was always interested in being some sort of scientist and experiences volunteering at a small aquarium, along with taking an conservation biology class in high school, fostered that interest. However, it was in my first evolutionary biology class, when I discovered population genetics, that I got excited about evolutionary biology. This enthusiasm led me to a research assistant position in an evolutionary ecology lab, where I got to participate in the often-tedious-but-occasionally-exciting business of actual science. After graduation, I worked as a technician studying speciation in wild tomatoes and rapid adaptation in Trinidadian guppies before starting graduate school at the University of Toronto. I spent my PhD trying to disentangle how selection and drive shape genetic variation in the weedy plant species Capsella grandiflora. I found that negative selection against new mutations and positive selection for new mutations shape broad patterns of genomic variation and that much of the genomic variation that actually affects gene expression is under negative selection. Now, as a postdoctoral researcher at the University of California, Davis, I am continuing to investigate the evolutionary forces shaping variation by studying if and how local adaptation contributes to quantitative trait variation in domesticated maize. My goal is to not only answer these questions in maize but to also develop methods that will be useful for investigating additional species and, overall, I hope that my work linking patterns of genomic variation with trait variation will contribute to a better understanding of how evolution shapes the world around us.
See Emily Josephs’ presentation abstract.
Emily C. Moore
North Carolina State University
One of my overarching research questions is ‘how do changes to the genome allow for the varied patterns of behavior we see reflected across the animal kingdom?’ I was inspired to start exploring this idea during my undergraduate study of biology and philosophy of mind, and I hope to continue to ask questions like these through the rest of an academic career. I have always been curious about how biological systems work, though it wasn’t until my early twenties that I recognized that a career in scientific research would be more engaging than a career in medicine—where I could contribute to the field of knowledge rather than merely absorb it. One of the focuses of my doctoral work has been to understand the genetic basis of behavioral differences between ecologically-varied cichlid fish species. I have been fortunate to be able to identify an interesting phenotype (an exploratory behavior), explore the genetic architecture of the behavior, and ultimately identify and evaluate a specific genetic variant within the time span of a PhD. The beauty of asking these questions in an ecologically and evolutionarily interesting vertebrate model organism is that our findings are relevant to the fields of evolutionary ecology and vertebrate neurobiology. The more we understand about how natural variation in the vertebrate genome shapes the development and function of the brain, the better insight we can have into how behavioral patterns evolve, and how disruption to neurogenetic pathways can lead to brain and behavioral dysfunction.
Katherine Xue
University of Washington
I never liked biology before I started learning about evolution. As a kid, I was fascinated by the order and rigor of math, but biology seemed like a chaotic mess. It wasn’t until high school that a teacher suggested I read books by Stephen Jay Gould and Richard Dawkins. Before long, I was hooked. I wanted to understand how evolutionary principles could unify and explain what Darwin called life’s “endless forms most beautiful.”
I started combining my quantitative leanings with my new love of biology. As an undergraduate, I bred tetraploid plants to understand how meiosis adapts to the tangled complications of having extra chromosomes. After graduating, I worked for a year as a science writer, inspired by the writers who first brought me to evolutionary biology.
As a graduate student, I study the lightning-fast evolution of influenza. Flu viruses change incredibly rapidly, requiring constant monitoring and vaccine updates. Recent advances in genome sequencing make it possible to track this evolution at high resolution. My work has shown that flu viruses can rapidly and repeatedly evolve to cooperate with one another in laboratory settings. I’ve also used new sequencing technologies to zoom in on how flu evolves in individual people while they’re sick. Surprisingly, I found that flu viruses can evolve in infected people over time in ways that mirror global evolution, which helps us understand better how flu evolution starts. I find it exciting and inspiring that evolutionary principles can shed light on important problems affecting human health.
Go to Katherine Xue’s presentation abstract.
Abstracts
Population Genetic Models for Complex Disease Evolution
Jeremy Berg, Guy Sella
Biological Sciences, Columbia University, New York, NY
A decade into the era of well powered and reproducible genome wide association studies, one thing is clear: many complex diseases are extremely polygenic, with thousands or perhaps tens of thousands of segregating variants contributing to variation in risk among individuals. However, our understanding of the reasons for variation among diseases in their prevalence, as well as in the number, frequencies, and effect sizes of mutations which contribute to variance in risk, is limited.
We construct and analyze a model of a highly polygenic complex disease at evolutionary equilibrium under mutation- selection-drift balance. In our model, disease arises due to a global epistasis among mutations which act additively on the liability scale (i.e. a liability threshold model). Selection occurs at the level of the disease phenotype, while selection coefficients experienced by individual loci arise as dynamic variables of the system. We show that in fact, so long as the disease is sufficiently polygenic, the selection coefficients of individual loci are insensitive to the fitness cost of the disease, and instead depend on the distribution of effect sizes and the degree of mutational bias toward increased disease liability. This result is robust to the assumption of a strict liability threshold, and also holds in the presence of some forms of pleiotropy. We also show that the results of genome wide association studies appear to be qualitatively inconsistent with mutation-selection-drift equilibrium, assuming modern prevalence and fitness cost of disease, but that pleiotropy and/or very recent environmental change can potentially explain these inconsistencies.
Intra-patient evolutionary dynamics of HIV drug resistance evolution in time and space
A.F. Feder1, Z. Ambrose2, R.W. Shafer1, S-Y. Rhee1, S. Holmes1, J. Hermisson3, P.S. Pennings4, D.A. Petrov1.
1) Stanford University, Stanford, CA; 2) University of Pittsburgh, Pittsburgh, PA; 3) University of Vienna, Vienna, Austria; 4) San Francisco State University, San Francisco, CA
At the beginning of the HIV epidemic, drug resistance to treatment evolved quickly and predictably across all patients. Now we treat HIV with combination therapies of three drugs so any single HIV mutation is insufficient for viral replication. The rate of drug resistance evolution has plummeted in response. Despite these advances, a minority of viral populations become resistant nonetheless. Why and how do certain populations overcome efficacious combination therapy? In answer to the first question, we analyze how the mode of drug resistance evolution changed within patients throughout the epidemic using historical HIV sequences. We find evolution has shifted from multiple origins of drug resistance (“soft sweeps”) to single origins (“hard sweeps”) as treatments have improved. This observation suggests that while drug resistance was once inevitable, now patients that fail combination therapy are merely unlucky, and not predestined to fail due to factors like poor adherence. However, questions remain about how hard sweeps of drug resistance can occur at all under combination therapy. Theory suggests that spatial structure of the intra-patient population drive multidrug resistance through creating pockets of spatial monotherapy whereby mutations can be acquired sequentially instead of simultaneously (Moreno-Gamez et al., 2015). However within-body population structure remains unknown. To bridge this gap, I analyze Simian-HIV-infected macaques sampled spatially and temporally during drug resistance evolution. We observe that populations from different organs (gut, plasma, lymph node, vagina) within the same macaque can be significantly different although the magnitude of the difference varies through time as drug resistance emerges. From these data, we quantify the population genetic parameters of the intra-patient environment to aid modeling efforts such as the spatial-monotherapy work. Notably, we develop a new ABC framework for estimation in adapting populations that can estimate migration rates much larger than those possible to estimate from tracking neutral alleles. This represents the first quantitative description (to our knowledge) of the within-patient spatial structure of HIV that accounts for adaptation.
A mechanistic model of assortative mating in a hybrid population
Amy Goldberg1,2, Noah Rosenberg2
1)Integrative Biology, UC Berkeley, 2)Biology, Stanford University
Mating pairs often exhibit levels of similarity in phenotypic or genotypic traits that differ systematically from the level expected under random mating, produced by assortative mating. For example, in admixed human populations, spouses possess correlated ancestry components suggestive of positive assortative mating on the basis of ancestry. Additionally, assortative mating has been proposed as a mechanism for hybrid and sympatric speciation. Using a two-sex mechanistic admixture model, we devise a model of preferential mating based on source population during hybridization. Under the model, we study the distribution of genetic ancestry on the autosomes and X chromosome for positive and negative assortative assortative mating, allowing migration to the hybrid population to vary between sexes and over time. We demonstrate that, whereas the mean admixture under assortative mating is equivalent to that of a randomly mating population, the variance of admixture generally increases with higher levels of positive assortative mating and decreases with negative assortative mating, analogous to classic theory on assortative mating by single locus genotypes or traits. However, perhaps contrary to previous work, we identify cases in which positive assortment can decrease the variance because mate preferences co-occur in multiple populations—the parental and hybrid populations. The effect of assortative mating is smaller on the X chromosomes than the autosomes because inheritance of the X in males depends only on the mother’s ancestry, not on the mating pair. As the variance of admixture has been used to infer the timing of hybridization and sex-biased admixture, we consider the implications of assortative mating for inferring population history and speciation. Our model provides a framework to quantitatively study assortative mating under flexible scenarios of mating and hybridization over time.
Detecting polygenic adaptation in maize
E. Josephs 1, 2, J. Berg3, J. Ross-Ibarra2,4, G. Coop1,2.
1) Department of Evolution and Ecology, University of California, Davis, Davis, CA; 2) Center for Population Biology, University of California, Davis, Davis, CA; 3) Department of Biological Sciences, Columbia University, New York, NY 10027, USA; 4) Department of Plant Sciences, University of California, Davis, Davis, CA
Characterizing the genetic basis of adaptation is not only a longstanding goal of evolutionary biology, but is also an important component of understanding adaptation. Adaptation in quantitative traits likely often occurs through subtle shifts in allele frequencies at many loci, a process called polygenic adaptation. Even though many traits have a polygenic basis, conventional methods lack power for detecting polygenic adaptation. In this talk, I describe strategies for detecting polygenic adaptation at the phenotypic and genotypic level. I show that we can leverage trait-associated loci identified from genome-wide association studies to detect the coordinated shifts in allele frequency expected under polygenic adaptation. Application of my methods to different maize populations shows evidence for polygenic adaptation in a number of traits in both inbred lines from the USDA germplasm pool and European landraces. Ultimately, these methods can be applied to multiple domesticated and wild species to give us a broader picture of the the specific traits that contribute to adaptation and the overall importance of polygenic adaptation in shaping trait variation.
Genetic variation at a conserved non-coding element contributes to microhabitat-associated behavioral differentiation in Malawi African cichlid fishes
Successful behavioral adaptation to habitat is fundamentally important to species fitness, but linking such behaviors to genes has proven difficult due to plasticity of phenotypes and rarity of within-species genetic variation underlying adaptive behavioral patterns. The East African cichlid fishes of Lake Malawi are ideal for investigating behavioral adaptation to environment, as within genera, fine-scale niche partitioning has resulted in sympatric sister species that live in definable microhabitats with distinct selection pressures. We tested species of Malawi cichlids found in rocky reefs, open sand habitats, or the sand-rock interface for a variety of environment-usage phenotypes in a controlled laboratory setting. Computer-aided analysis of fish response to new environments revealed distinct behavioral patterns among sand, rock and interface species. In the lab, we created a hybrid cross between two genera that differ in microhabitat in the wild and behavior in the lab, and used a ddRAD-seq linkage-mapping strategy to identify quantitative trait loci (QTL) associated with species-specific behaviors. Comparative genomic analysis from 82 wild and wild-derived species grouped by microhabitat-use identified variation corresponding with one of these QTL, further supporting broad association with habitat use across the Malawi cichlid radiation. This locus contains a conserved non-coding element (CNE) upstream of three neuronal cell adhesion molecule (NCAM) genes, where the derived “sand” allele appears to disrupt a neuronal transcription factor binding motif. Additionally, we used interface species that were naturally segregating the “rock” allele and “sand” allele at the NCAM CNE to confirm that genotype at the locus is associated with behavioral variation among full siblings within a species. Finally, allele-specific expression indicates that expression of one of the three NCAM genes has a two-fold reduction in expression when linked to the derived “sand” allele at the CNE. Together, these integrated results suggest that evolution of gene expression at the NCAM locus has accompanied behavioral adaptation to microhabitat, which could ultimately reinforce speciation through spatial isolation.
Evolutionary dynamics of influenza across spatiotemporal scales
K.S. Xue1,2, T. Stevens-Ayers3, A.P. Campbell3, J.A. Englund4,5, S.A. Pergam3,6,7, M. Boeckh3,6,7, J.D. Bloom1,2.
The rapid global evolution of influenza viruses begins with de novo mutations that arise in individual infected hosts. Recent advances in high-throughput deep sequencing have made it increasingly possible to measure influenza’s within-host genetic diversity and compare this diversity to the virus’s global evolution. We demonstrate that influenza evolution within infected humans recapitulates many evolutionary dynamics observed at the global scale. We deep-sequence longitudinal samples from four immunocompromised patients with long-term H3N2 influenza infections. We find parallel evolution across three scales: within individual patients, in different patients in our study, and in the global influenza population. In the viral surface protein hemagglutinin, a small set of mutations arises independently in multiple patients. These same mutations emerge repeatedly within single patients and compete with one another, providing a vivid clinical example of clonal interference. Many of these recurrent within-host mutations also reach a high global frequency in the decade following the patient infections. These results demonstrate that influenza viruses can evolve rapidly in chronic infections in ways that mirror global viral evolution. We close by discussing major open questions about how genetic drift, purifying selection, and positive selection combine to shape influenza’s evolution within hosts during more typical, acute infections, and how this within-host diversity contributes to the virus’s global evolution. Altogether, our analyses illuminate how evolutionary forces act on viral populations across interlocking scales of space and time.